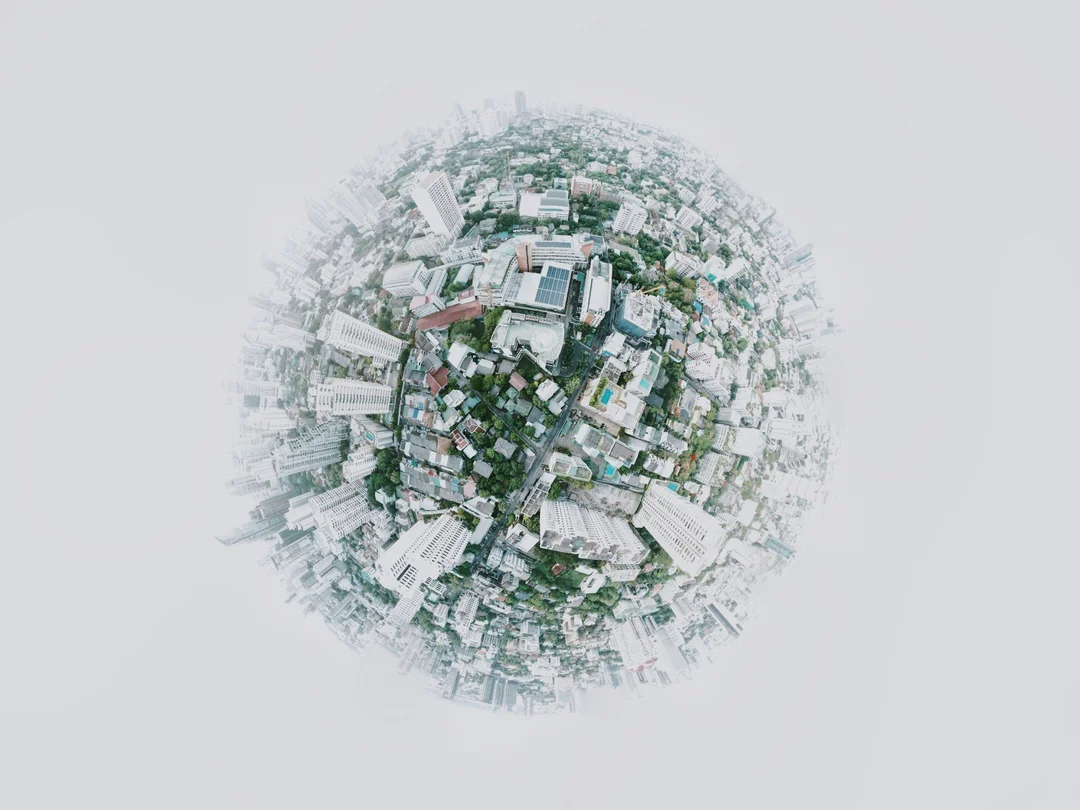
Black Hole Collisions Reveal Deep Connection to String Theory’s Calabi-Yau Manifolds
In a groundbreaking advancement, physicists have discovered a surprising link between black hole scattering and the abstract mathematical concepts of string theory. A new study published in Nature unveils that the interactions of black holes and neutron stars, specifically their near-miss encounters, can be accurately modeled using Calabi-Yau manifolds, intricate geometric structures originating from string theory. This finding bridges the gap between theoretical physics and observable astrophysical phenomena.
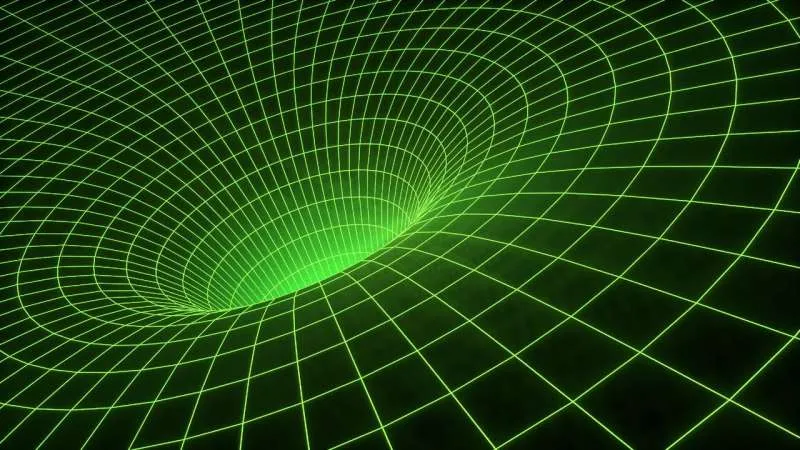
The research, led by Professor Jan Plefka at Humboldt University of Berlin and Dr. Gustav Mogull at Queen Mary University London, achieves an unprecedented level of precision in modeling the universe's most extreme events. They calculated the fifth post-Minkowskian (5PM) order for observables related to black hole collisions, including scattering angles, radiated energy, and recoil. The unexpected appearance of Calabi-Yau three-fold periods within the radiative energy and recoil opens new avenues for understanding gravitational waves.
Gravitational waves, ripples in spacetime caused by accelerating massive objects, have revolutionized astrophysics since their first detection in 2015. As gravitational wave observatories like LIGO become increasingly sensitive, and with next-generation detectors such as LISA on the horizon, theoretical models of exceptional accuracy are crucial. Dr. Mogull emphasizes the mathematical and computational precision needed to study these conceptually simple yet intensely complex interactions involving black holes.
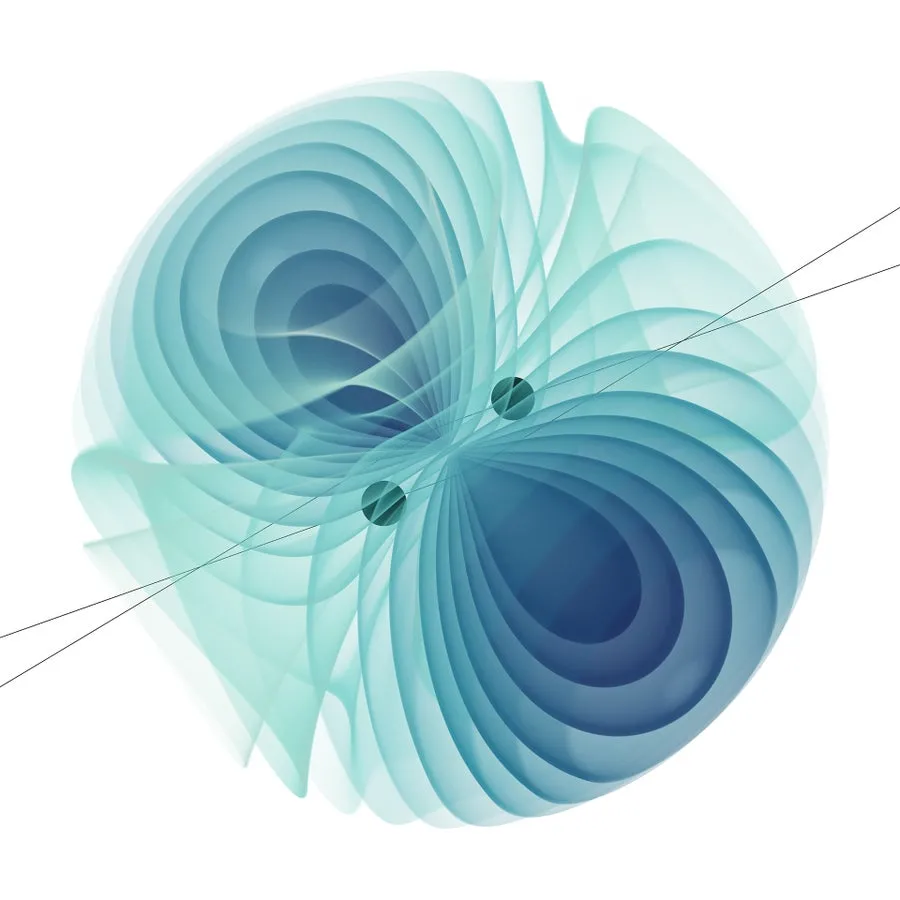
Benjamin Sauer, a Ph.D. candidate at Humboldt University of Berlin, notes the profound implications of Calabi-Yau geometries. These insights will advance gravitational wave astronomy by refining the templates used to interpret observational data, enabling precise capture of signals from elliptic bound systems where orbits closely resemble high-velocity scattering events.
The discovery of Calabi-Yau structures in this context highlights the interconnectedness between the macroscopic realm of astrophysics and the intricate mathematics of quantum mechanics. Dr. Uhre Jakobsen of the Max Planck Institute for Gravitational Physics states that this could fundamentally change physicists' approach to these functions, as it demonstrates their physical relevance and allows researchers to focus on specific examples that illuminate genuine processes in nature.
Using over 300,000 core hours of high-performance computing at the Zuse Institute Berlin, the team tackled the complex equations governing black hole interactions, underlining the indispensable role of computational physics in modern scientific inquiry. The swift availability of these computing resources was key to the success of this project, according to Ph.D. candidate Mathias Driesse, who spearheaded the computing efforts.
This breakthrough not only enriches gravitational wave physics but also closes the gap between abstract mathematics and the observable universe. Professor Plefka calls it a testament to how interdisciplinary efforts can overcome challenges once considered insurmountable. The expanded collaboration plans to explore higher-order calculations and apply the new results in future gravitational waveform models. Moreover, computational tools like KIRA, used in this study, could also benefit fields such as collider physics.
The future of gravitational wave astronomy looks bright, as these newly discovered connections and advanced modeling techniques pave the way for even more profound cosmic discoveries. The ability to discern between catastrophic collisions versus near-miss events opens a window into the evolutionary path of black holes in ways previously unavailable.
What are your thoughts on the implications of string theory influencing real-world astrophysics? Share your insights and questions in the comments below!